EnVision at Venus: Europe’s next Medium-class science mission
June 10, 2021: EnVision selected by ESA
On June 10, 2021, the European Space Agency (ESA) announced the selection of EnVision as its newest medium-class science mission. ESA’s Solar Orbiter, Euclid, Plato and Ariel have already been selected as Medium-class missions. EnVision will make detailed observations of Venus to understand its history and especially understand the connections between the atmosphere and geologic processes. As a key partner in the mission, NASA provides the Synthetic Aperture Radar, VenSAR. EnVision will deliver new insights into Earth’s nearest planet’s geological history through complementary imagery, polarimetry, radiometry and spectroscopy of the surface coupled with subsurface sounding and gravity mapping; it will search for thermal, morphological, and gaseous signs of volcanic and other geological activity; and it will trace the fate of key volatile species from their sources and sinks at the surface through the clouds up to the mesosphere.
EnVision Science Payload
EnVision’s science payload consists of VenSAR, a dual polarization S-band radar also operating as microwave radiometer, three spectrometers VenSpec-M, VenSpec-U and VenSpec-H designed to observe the surface and atmosphere of Venus and their couplings, and the Subsurface Radar Sounder (SRS), a High Frequency (HF) sounding radar to probe the subsurface. These are complemented by a radio science investigation which achieves gravity mapping and radio occultation of the atmosphere, for a comprehensive investigation of the Venusian interior, sub-surface, surface, lower atmosphere, cloud level, upper atmosphere properties and their interactions. This suite of investigations works together to comprehensively assess surface and subsurface geological processes, interior geophysics and geodynamics, and atmospheric pathways of key volcanogenic gases, which together illuminate how and why Venus turned out so differently to Earth.
EnVision Science Objectives
Venus History
Venus accounts for 40% of the mass of terrestrial planets in our solar system, yet even fundamental parameters such as the relative size of its core to mantle are unknown. As we expand the scope of planetary science to include those planets around other stars, the lack of measurements for basic planetary properties such as moment of inertia, whether parts of its current surface was formed in the presence of water, core-size and density variations with depth, and thermal profile for Venus hinders our ability to compare the potential uniqueness of the Earth and our solar System to other planetary systems.
Left : a 225 meter per pixel Magellan radar image mosaic of Venus, centered at 47 degrees south latitude, 25 degrees east longitude in Lada regio. The scene is approximately 550 kilometers (341 miles) east-west by 630 kilometers (391 miles) north-south. The mosaic shows a system of east-trending radar-bright and dark lava flows encountering and breaching a north-trending ridge belt (left of center). Upon breaching the ridge belt, the lavas pool in a vast, radar-bright deposit, covering approximately 100,000 square kilometers (right side of image). The source caldera for the lava flows, named Ammavaru, lies approximately 300 kilometers west of the scene (JPL/Caltech). Right : at latitude 6.6 south , and an east longitude 298.6 degrees, lies this 100.5 km wide volcano.
Venus Activity
Given its similar size and bulk composition as Earth, Venus is expected to be volcanically and tectonically active today (unlike Mars, which, being only a tenth of Earth’s mass, has lost more of its internal heat). Steep slopes and landslides are common on Venus’ surface geology, implying active uplift, but existing data provide no constraint on current rates of tectonic activity. Comparaison of gravity mapping and topography have revealed some regions which appear to be at higher elevation than would be the case if the mantle were stagnant, implying that they lie atop magmatic upwelling. There are regions where volcanic activity is particularly likely to occur. Sites of potential volcanism identified in Venus Express data were in these locations. Therefore the observation and characterisation of these regions are of high priority.
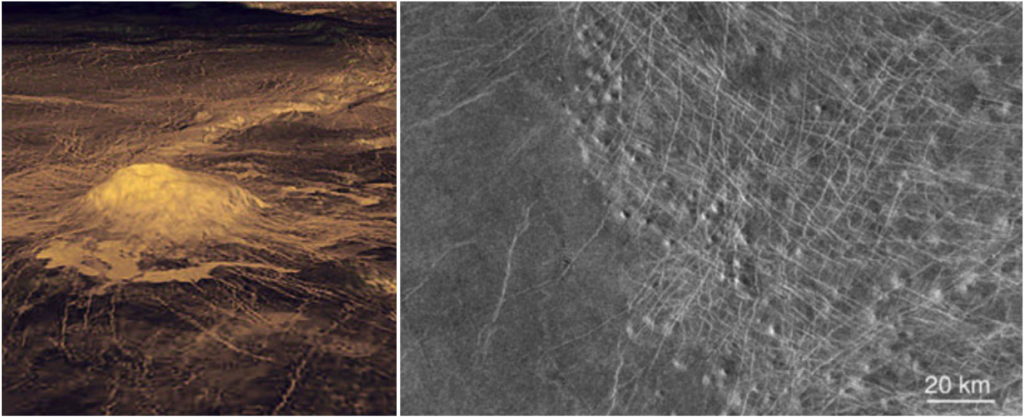
Left : Idunn Mons (46 S; 146 W) in Imdr Regio, with its steep-sided dome at the summit, as well as lava flows extending in the surrounding plain, is characterised with high emissivity measurements pointing to recent volcanism; right : a volcanic shield field inside a corona structure, surrounded by a set of younger lava plains. At all scales, there is a huge variety of unexplained features on Venus – from volcanic, tectonic features (e.g. wrinkle ridges), evidence of large flows (canali), aeolian features that we do not understand in terms of a basic sequence of events. Are canali or other specific magmatic features confined to a past regime or still active today? Is there a correlation between mesospheric SO2 concentration and volcanic activity? On Venus, the spatial distribution of impact craters cannot be distinguished from a spatially random population. This indicates a much younger surface than most of the Earth’s continents (less than 600-800Ma).
Venus Climate
What is the clouds relationship to long-term climatology and active volcanism?
The high-resolution images of Venus’ cloud deck acquired a decade ago by the Venus Monitoring Camera on board Venus Express showed, as never before, the details of convective structures and gravity waves, and how crucial those small-scale phenomena are for understanding the Venusian climate. Our current understanding of the clouds suffers from the limitations of the available data (very little in situ observations are available within the cloud layers), and the lack of complete models to interpret the observations. One- and two-dimensional modeling studies on Venus clouds have been published since late 1990s (James et al., 1997; Imamura and Hashimoto, 1998, 2002), one advanced cloud model has been extensively used and developed further to study the clouds and interpret the data (McGouldrick & Toon, 2007, 2008a, 2008b). Latest microphysical models include all the main microphysical processes: homogeneous nucleation of sulfuric acid solution particles (Määttänen et al., 2018), heterogeneous nucleation through a simple parameterisation (James et al., 1997), condensation/evaporation processes and Brownian coagulation (Pruppacher & Klett, 1997). The clouds of Venus play a large role in atmospheric chemistry (serving as reservoirs of water and sulphur in the form of sulphuric acid), radiative balance (reflecting away 70% of the light falling on Venus, and absorbing much of the rest), and therefore also in the atmospheric dynamics. In the 45-65 km altitude range, sulfur and water chemical cycles are coupled through the formation of H2SO4-rich cloud droplets. EnVision’s investigation will focus on understanding the chemical cycles and transport of SO2, H2O and H2SO4 involved in cloud forming processes.
How are interannual variations of mesospheric SO2 linked to volcanic processes?
The interannual variations of mesospheric SO2 – sharp rises followed by gradual declines in following years – are suspected as one of the potential signs of active volcanism (Esposito, 1984, Marcq et al., 2013). AThese enhancements are associated with increased vertical mixing rather than direct volcanic injection. EnVision will carry out mapping of SO2, H2O and related compounds both below and above the clouds, to characterise as extensively as possible (wrt. latitude, local solar time, longitude) their spatial and temporal distribution, and thus help in determining whether at least some of these increases in vertical mixing are caused by transient thermally buoyant volcanic plumes.
EnVision : Complementary Investigations
EnVision will use a number of different techniques to search for active geological processes, measure changes in surface temperature associated with active volcanism, characterise regional and local geological features, explore the lower and upper atmosphere, determine crustal support mechanisms and constrain mantle and core properties :
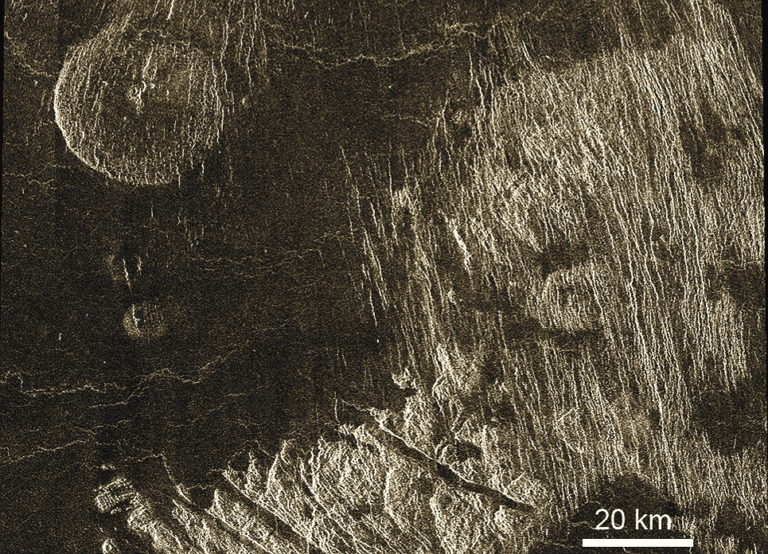
Densely fractured plains (image right, cut by NNW set of faults) embaying tessera (bottom, cut by multiple sets of faults) indicating that emplacement of the material of the densely fractured plains postdated formation of tessera terrain. Both densely fractured plains and tessera are embayed by even younger regional plains (dark areas). A steep-sided volcanic dome is seen in the upper left. Its age relations with the surrounding regional plains are not known. Portion of Magellan image centered at 46N, 360E. After Basilevsky and McGill (2007), Surface evolution of Venus, in Exploring Venus as a Terrestrial Planet, Geophysical Monograph Series 176, edited by L. W. Esposito, E. R. Stofan and T. E. Cravens, pp. 23-43, American Geophysical Union, Washington, DC.
- Use differential interferometric SAR (DiffInSAR) to look for cm-scale surface changes over large areas. This can detect volcanic changes such as new lava flows, or for possible inflation or deflation of underground magma chambers;
- ?Search for changes in repeated radar imagery of regions of interest. Change detection can be achieved either by looking for changes in reflection properties, or from changes in unit boundaries;
- Look for thermal signature of volcanic activity. This can be done through repeated observations either in near-IR spectral window regions and in microwave wavelengths;
- Spectrometry of different surface units is critical for understanding their composition. Although the optically thick Venus atmosphere precludes this at most wavelengths, there are five spectral windows between 0.8 and 1.2 μm at which thermal emission from the surface escapes to space. Surface emissivity at these wavelengths encodes crucial information about mineralogical characteristics like Fe content. Emissivity mapping helps to test theories of surface composition, for example about whether the tessera highlands are composed of felsic materials akin to Earth’s granitic continental crust, and therefore were formed in a volatile-rich environment;
- Search for plumes of possibly volcanogenic gases such as H2O, SO2, CO or OCS, reflecting current and active volcanic activity;
- Sensitivity to unit boundaries beneath the surface, and ability to detect buried structures, would significantly enhance the reconstruction of stratigraphy and thus in the reconstruction of the geological record. Subsurface sounding can enable measurement of the depth of volcanic flows and therefore the volume of volcanic effusions; of buried unit boundaries beneath volcanic plains, such as mapping of the edges of tessera regions; and could reveal unexpected features such as buried impact craters;
- The internal properties of Venus. Venus is less dense than expected if it had similar Earth’s bulk composition. In order to assess information on its interior structure, it is crucial to determine the k2 Love number and the moment of inertia. The tidal Love number, estimated from Doppler tracking of Magellan and Pioneer Venus Orbiter spacecraft data, is not accurate enough to constrain the state and size of the core, the mantle composition and viscosity. EnVision shall map the gravity field for at least 50% of the surface with spatial resolution of better than 200 km, that is equivalent to the knowledge of spherical harmonics to at least degree of 90 and accuracy of at least 10 mGal/km. EnVision will also measure the k2 Love number of the planet with an accuracy better than ±0.01 –compared to its current knowledge to ±0.066 uncertainty – allowing to constrain the size and state of Venus’ core and the composition of Venus’ mantle.
More information on the EnVision mission website